Catalysis to Deliver Polymers from Carbon Dioxide
Carbon dioxide is well-known to be hard to activate and its chemistry is constrained by high thermodynamic stability and slow kinetics. Whilst small-scale, stoichiometric transformations are possible, truly catalytic processes feasible for use at scale are very rare. One such reaction is carbon dioxide and epoxide copolymerisation to form polycarbonates. This reaction results in a triple win in carbon dioxide emissions savings: for every molecule of carbon dioxide take up in the polymer backbone, two more are saved by avoiding the use of the petrochemical (epoxide). Both the process viability and polymer applications depend upon the selection of the polymerisation catalyst. Our team have developed a series of metallic catalysts, the best of which combine high activity, selectivity and operability under low CO2 pressures, including at 1 bar.
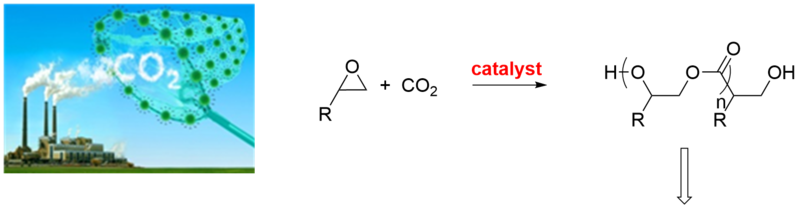
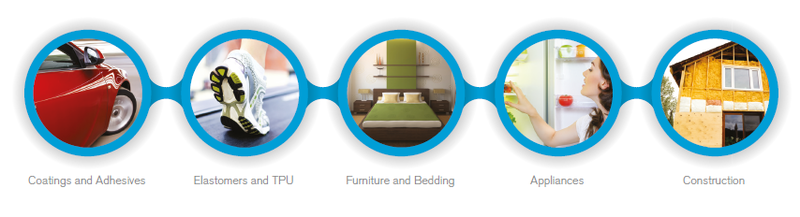
In 2008, we reported di-Zn(II) catalysts which showed good activity at 1 bar pressure of carbon dioxide, yielding perfectly alternating polycarbonates. In the years since, we have focussed on improving both the fundamental understanding and absolute catalytic performances of these catalysts. One potential benefit of these low-pressure catalysis is their suitability for retro-fit of existing manufacturing plant. These catalysts and processes deliver polymers useful for sustainable energy generation, as surfactants, in automotive or soft-furnishing foams, and in home-insulation sectors, amongst others. We are actively involved in investigating the polymer chemistry and application potential for these aliphatic polycarbonates.

My research team focus on new catalyst development and understanding of catalytic performances. We have been inspired by metalloenzyme active sites and have investigated catalysts that deploy two metals, working cooperatively, to accelerate carbon dioxide activation. To understand their interesting properties, we investigate aspects such as the polymerisation kinetics, catalyst structure activity relationships, use spectroscopy to characterize catalytic cycle intermediates and, in collaboration, DFT models of the catalysis. Combining these findings has underpinned the ‘Chain Shuttling Mechanism’ whereby the polymer chain ‘shuttles’ between the two metals during propagation, with each metal playing a defined role in the catalytic cycle. We have investigated many different metals in these catalysts, including earth-abundant metals such as Na(I), K(I), Mg(II) and Fe(III). Many of these catalysts remain active even when water, or alcohols are added as chain transfer agents. Adding water provides a straightforward means to reproducibly deliver monomodal polymer molar mass distributions and to select for desirable end-groups, e.g. hydroxyl telechelic polycarbonates. Some catalysts remain active even when exposed to impure CO2 gas streams, including those deliberately contaminated with O2, CO, H2S, amines, H2O and N2. We showed that efficient and effective polycarbonate production was feasible when using CO2 captured from Ferrybridge power station.
Recently, we have been investigating heterometallic catalysts, some of which are synergic. For example, the Mg(II)/Zn(II) catalysts show better performances, under identical conditions, than either the di-zinc or di-magnesium catalysts or any combinations of them. To make and study these heterometallic catalysts has required exploration of their organometallic and coordination chemistry: in many cases the heterodinuclear complexes are thermodynamically more stable than homodinuclear analogues. Using the newly developed heterometallic syntheses we have made complexes combining different metals from Groups 1-13, including most first row transition metals. In 2020, we reported a Mg(II)/Co(II) complex which showed an activity of >12,000 h-1 with quantitative CO2 uptake; it was also synergic. In depth kinetic studies reveal it has a lower transition state entropy and enthalpy than either of the homodinuclear catalysts (Mg/Mg or Co(II)/Co(II)) and different roles for the two metals, with the Mg(II) centre reducing the transition state entropy whilst the Co(II) site reduces the transition state enthalpy. Such insights into synergy are rare but could also be relevant to other processes operating with a second metal additive or promoter. In 2020, we reported a new class of heterodinuclear catalysts and the Co(III)K(I) complex showed high activity, control and selectivity in alkylene oxide/CO2 ROCOP, delivering both hydroxyl telechelic poly(propylene carbonate) polyols and high molar mass PPC.
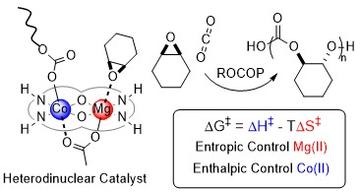
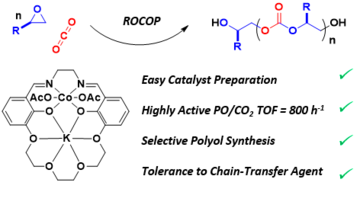